Cell Biology of Archaea
Our model organisms are the anaerobic hyperthermophilic Thermococcales archaea whose natural habitat is the oceanic hydrothermal vents. These archaea are able to multiply at an astonishing rate in this extreme environment. This remarkable adaptation is the basis of our work. Our team has a long documented experience in molecular genetics, protein biochemistry, genomics and bioinformatics. Our laboratories are equipped with all the latest technologies and equipment adapted to the culture of these extremophilic microorganisms. Recently, substantial progress has been made in the genetics of Thermococcales: it is now possible to easily modify their genome. Using a multidisciplinary approach, our research focuses more particularly on the plasticity of the genome of these organisms, the modalities of their gene expression and the relations of these archaea with the mineral world.
1. Plasticity and evolution of the Thermococcal genome
The Thermococcales archaea have relatively small genomes (~2Mb) which corresponds to half the genome of the model bacterium Escherichia coli. Our work has led us to sequence a large number of species and we now have more than 100 fully sequenced and annotated genomes. These circular genomes show a very high plasticity even if the essential genes are always located at a constant distance from the single origin of replication. We observed that these genomes evolve rapidly, even under laboratory conditions, through a particular recombination mechanism associated with the presence of mobile genetic elements. Our results have demonstrated that a particular class of integrases is able to generate homologous recombination events on these genomes. We are currently characterizing biochemically this new genomic recombination process. Following this discovery, we are interested in the properties, distribution and classification of archaeal integrases. This work contributes to decipher the molecular mechanisms of genome evolution in all living beings.
2. Gene expression in Thermococcales
Chromosomal DNA supercoiling is now recognized as an important epigenetic marker for the control of gene expression but also for DNA replication and repair. Deregulation of DNA over-winding homeostasis occurs in cancers, neurodegenerative diseases and some autoimmune diseases, however, the underlying molecular mechanisms are still poorly understood. Archaea are particularly interesting models to study DNA topology, as most of them have histones and DNA replication and transcription mechanisms homologous to those of Eukaryotes, but in a less complex cellular context and therefore easier to dissect. We are currently developing a technique to map DNA supercoiling in vivo at the genome scale in archaea. This technique should help us understand the role of DNA supercoiling in controlling gene expression and chromosomal DNA stability, particularly in hyperthermophilic archaea whose optimal growth temperature is above 80°C. Our ultimate goal is to understand how DNA supercoiling homeostasis is achieved in archaea and how it contributes to the overall 3D architecture of archaeal chromosomes.
tRNA molecules play a central role during the translation process and undergo numerous post-transcriptional modifications to ensure optimal and faithful decoding of mRNA. We are studying the biosynthetic pathway of one of the universal modifications of tRNA, called t6A (N6-threonylcarbamoyl adenosine). This modification adjacent to the anticodon is essential for the efficiency and fidelity of translation. The loss of this modification also generates various phenotypes such as severely impaired cell growth and increased sensitivity to DNA damaging agents in both prokaryotes and eukaryotes. In addition, mutations in t6A biosynthesis genes have recently been implicated in a severe human genetic disease called GAMOS. We use biochemical, proteomic, structural and genetic approaches to study the functions and evolution of the t6A biosynthetic pathway in Archaea. This work should contribute to a better understanding of the molecular basis responsible for the observed phenotypes, including in human diseases.
3. Biomineralization of Thermococcales
Abundantly found within hydrothermal vents called “black smokers”, Thermococcales interact with this extreme environment, characterized by strong physicochemical gradients and mainly composed of iron sulfide minerals such as pyrite (FeS2). While pyrite is formed abiotically inside the chimneys at high temperatures (> 250°C), a large stock of FeS2 is also produced at lower temperatures (< 150°C) by a still unknown mechanism. We have recently shown, that Thermococcales are able to rapidly produce abundant amounts of iron sulfides such as pyrite and greigite (Fe3S4). The sulfur vesicles produced by Thermococcales during cell growth [2] seem to be strongly involved in the biomineralization process and thus in the nature of the bio-minerals formed. This newly discovered biomineralization mechanism could be a strategy set up by Thermococcales to survive/adapt in their highly mineralizing extreme environment. In the laboratory, we are studying the physicochemical parameters and molecular mechanisms that influence the formation and nature of iron sulfide minerals produced by Thermococcales in order to better understand the role of Thermococcales in the biogeochemical cycles of iron and sulfur and thus allow the identification of new biosignatures of hyperthermophilic archaea within hydrothermal samples.
4. Bioinformatics of prokaryotic genomes
For several years we have been developing software in the form of web services for the manipulation and analysis of genomic sequences of the 50000 prokaryotic chromosomes completely sequenced, maintained locally and updated daily. The BAGET service allows to easily obtain the sequence and the immediate environment of any gene from these organisms. FITBAR allows the prediction of regulons, at the scale of a complete genome, based on specific sites provided by the user. SYNTTAX allows synteny analysis based on the taxonomic classification of different species. WASPS provides a fully resolved synteny approach for all known plasmids including user supplied sequences.
BAGET 2.0: Under development.
team
Violette DA CUNHA
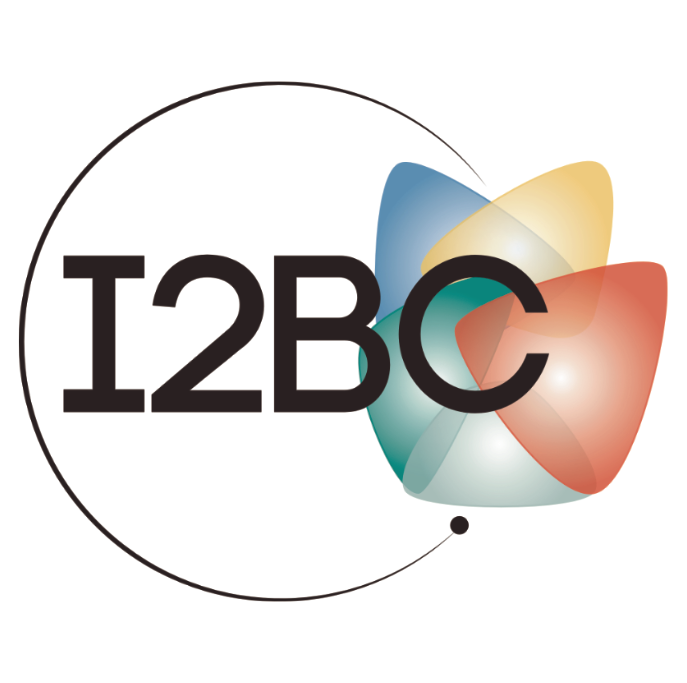
Researcher
Marie-Claire DAUGERON
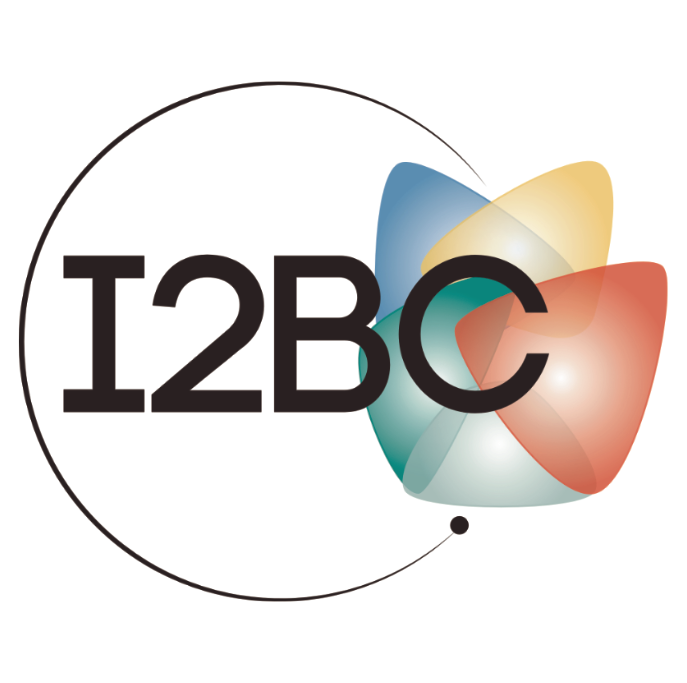
Researcher
Patrick FORTERRE
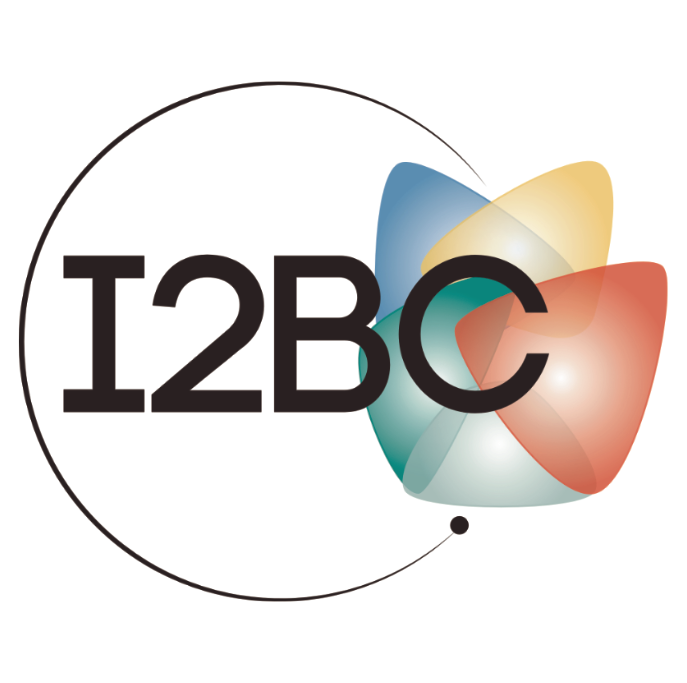
Emeritus Researcher
Tamara BASTA-LE BERRE
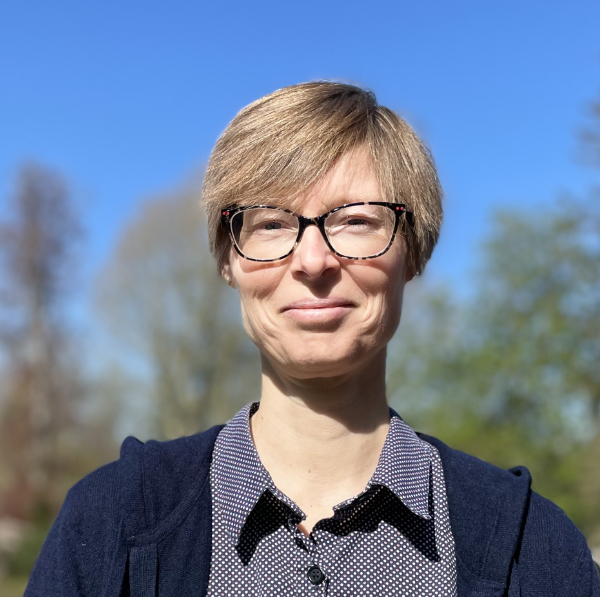
Researcher
Aurore GORLAS
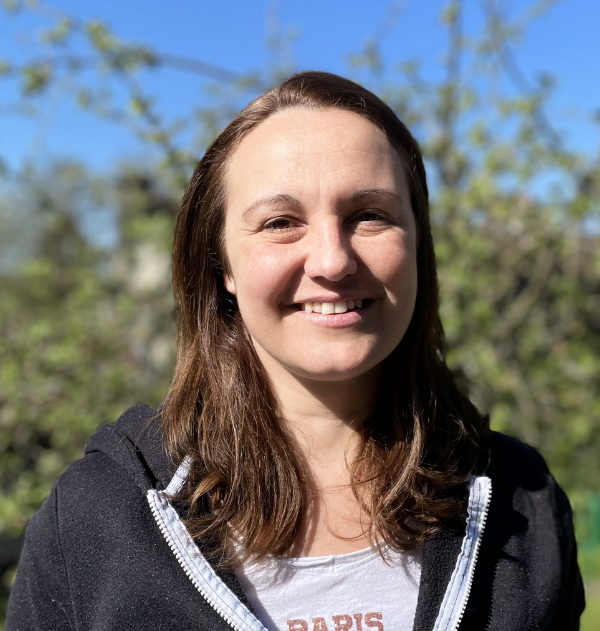
Researcher
Benjamin HEPP
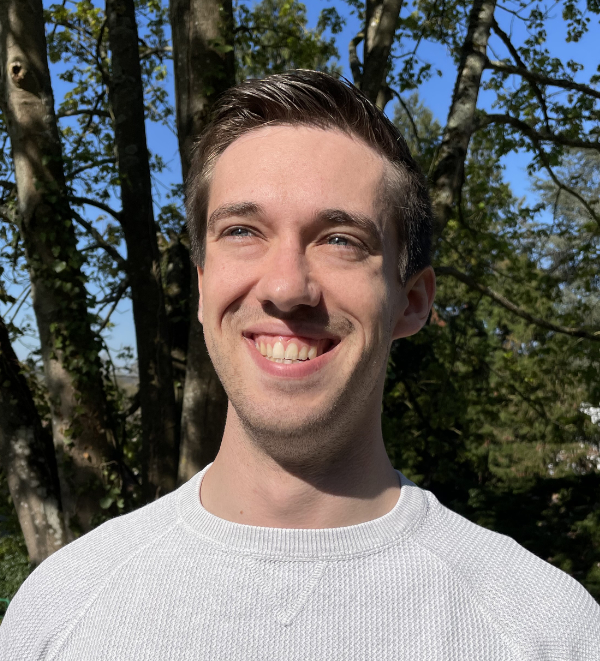
Phd
Tom MARIOTTE
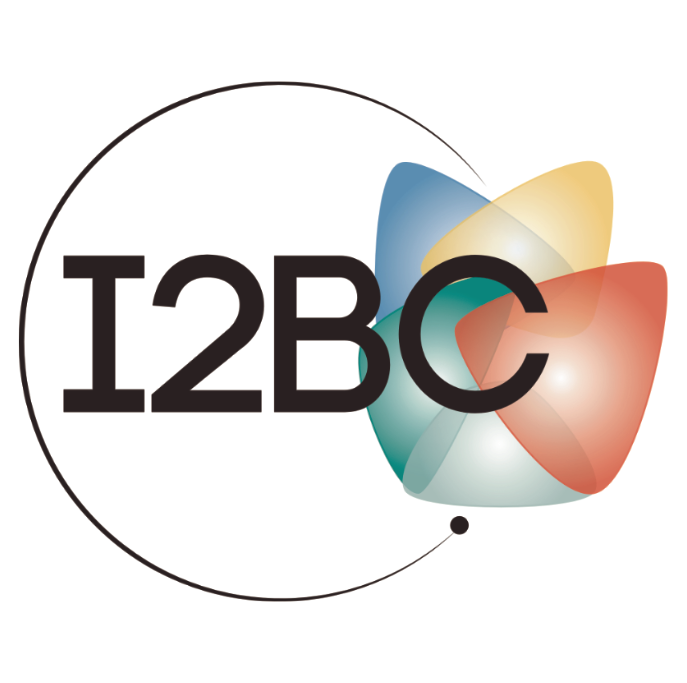
Phd
Mehdi ABDELLI
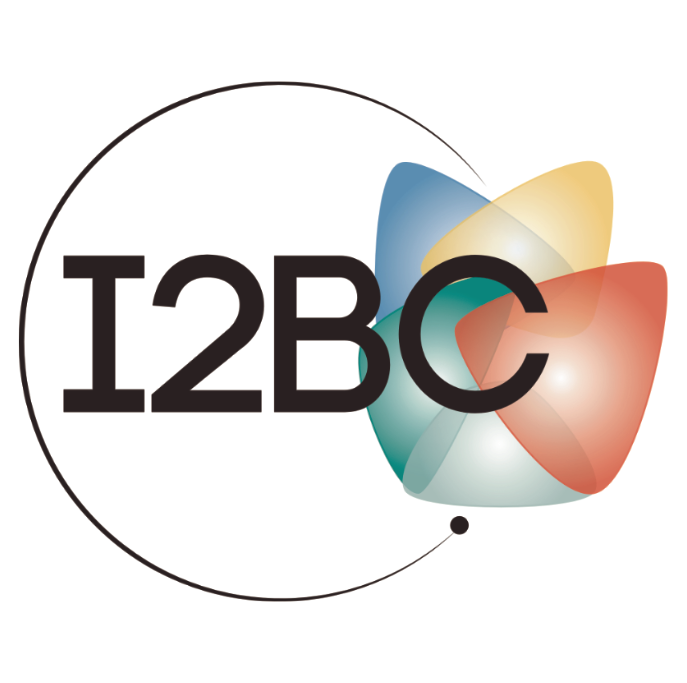
Phd
Florence LORIEUX
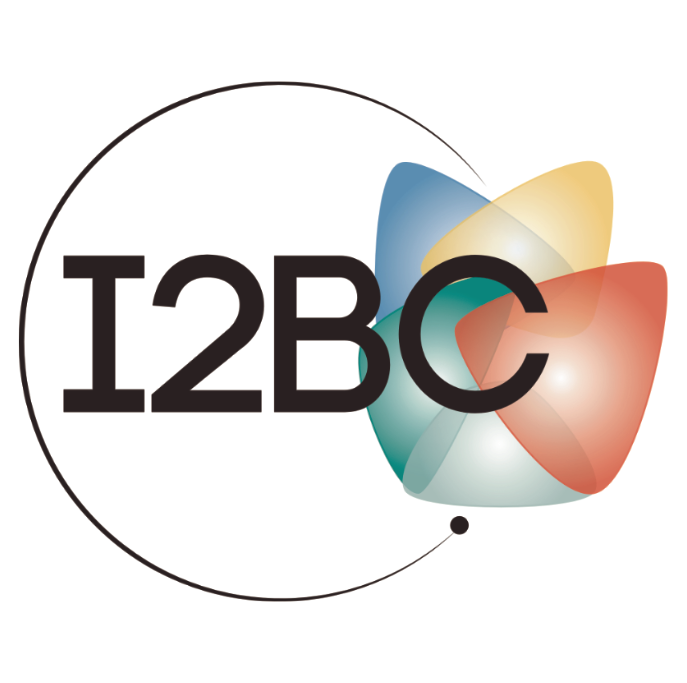
Technician
Augustin DEGAUGUE
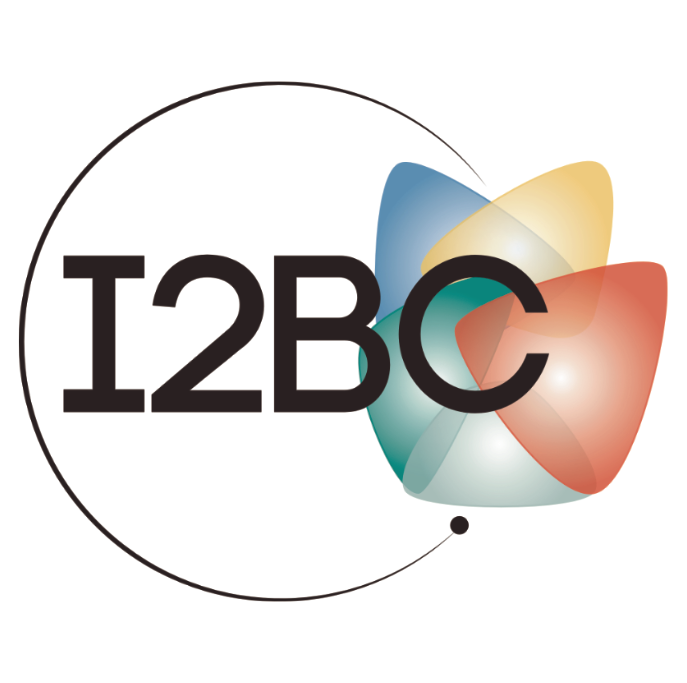
Technician
Solène LELEUX
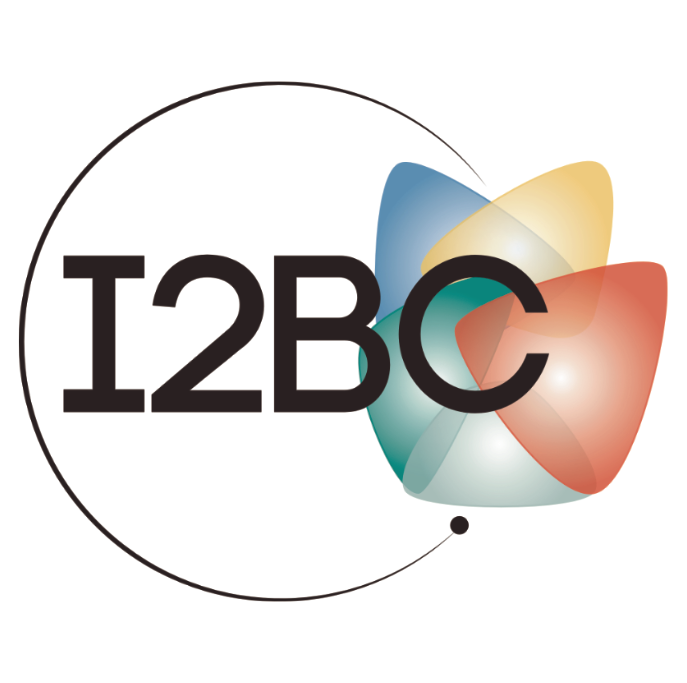
Master Intern
Romain COUDRAY
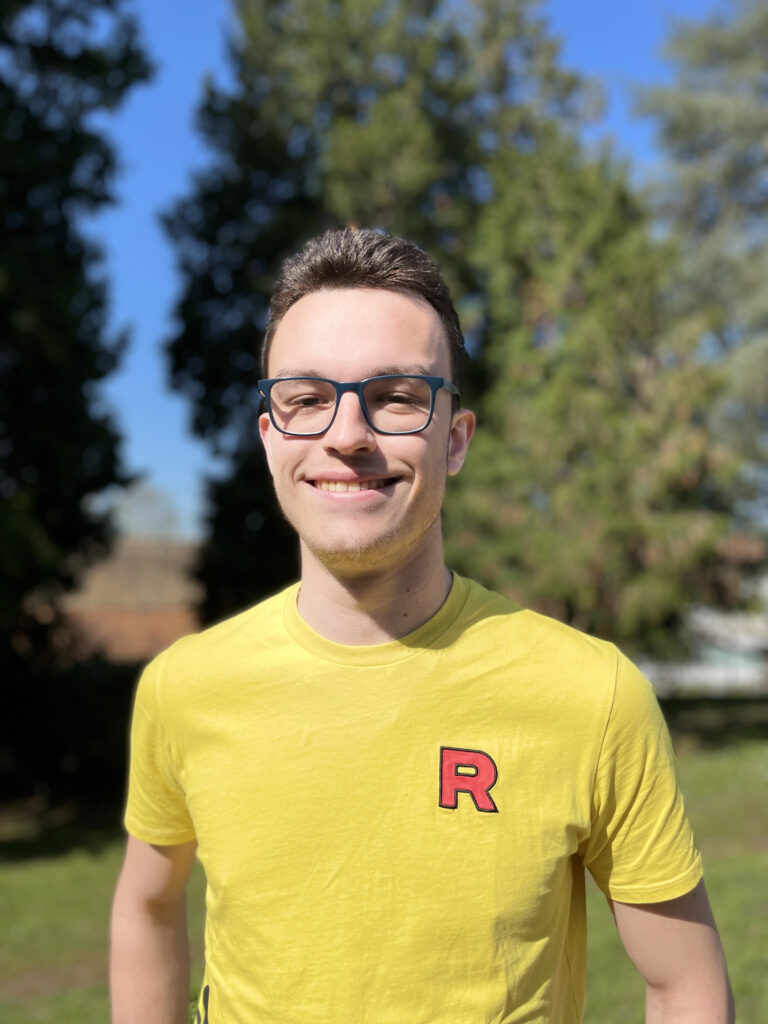
Master Intern
Sharleen BORG
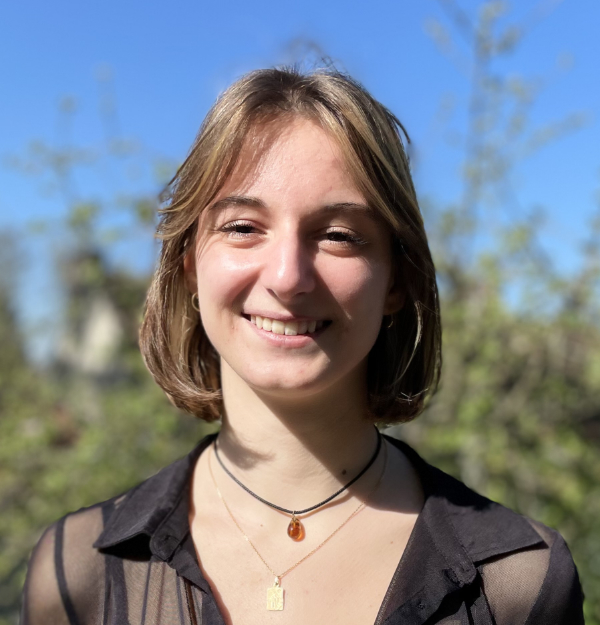
Intern Student
Latest publications
For all the publications of the Team click on the button below.